Fifty years ago, nuclear energy was part of any futurist’s vision of civilization.
Then came the Three Mile Island meltdown in 1979, when a Pennsylvania reactor failed, terrifying the American public. Construction of 52 nuclear reactors was canceled between 1980 and 1984. It did not help when in 1986 the Chernobyl disaster sent a cloud of radioactive smoke over eastern Europe. Most recently, in 2011, a tsunami hit Japan disabling the cooling system at the Fukushima plant. Public enthusiasm for nuclear power seemed to be in permanent decline.
Now, there is a looming energy shortfall caused by population growth, our ultra-technologized lifestyles, and ravenous data centers increasingly using artificial intelligence that can’t be built fast enough. (An AI-enabled Google search uses 10 times as much electricity as a normal Google search.) This projected shortage has many leaders endorsing an “all-of-the-above” approach to energy. And there, “above,” sits once-promising nuclear power.
It gets so little mention — the energy conversation being dominated by fossil fuels and renewables — that many Americans would be surprised to learn that 20% of electric power nationwide comes from nuclear plants. In Texas, due to the higher rates of coal and wind usage, the fraction is 10%. (That power comes from two plants: the South Texas Project outside Bay City and the Comanche Peak Nuclear Power Plant at Glen Rose.)
But that percentage could go way up if new research by a consortium relying on UT expertise is successful. The goal is to build reactors that would be very safe, small and therefore much more affordable to build, and modular, so individual users such as data centers or refineries might have their very own nuclear power plants yielding plentiful, steady electricity. What’s more, these new reactors could desalinate seawater or groundwater and help treat cancer by generating the isotopes necessary for treatment.
But first things first. And that’s research.
“What’s the potential for nuclear energ—”
“It could change the world,” says Derek Haas before the question is even completed. At UT, Haas, an associate professor of mechanical engineering, is leading the push to develop molten salt reactors. He believes these refrigerator-sized reactors would address the two things holding nuclear power back: public perception of nuclear safety, and cost.
***
Safety, first. Haas rejects the question of whether molten salt reactors would make nuclear power safe, because he says it always has been safe. “It’s always been statistically safer than any other form of energy, including renewables, when you factor in work accidents and transportation accidents.”
Moreover, he says, “When people think of Three Mile Island, Chernobyl or Fukushima, most of what they are thinking is wrong.”
- At Three Mile Island, no one died or had adverse health impacts from the release of radioactive material, he says. “People were justifiably scared because of the way it was handled, but the release of radioactive material did not harm anyone,” says Haas.
- Chernobyl on the other hand, was “as bad as nuclear can get because it was a really poor design operated with bad controls. People died, and that is absolutely something that we never want to happen again. Reactors today are designed, and in the United States they’ve always been designed, to avoid those kinds of impacts.”
- In Fukushima, the Japanese government paid out claims to one of the workers who was exposed to elevated levels of radiation voluntarily by trying to help alleviate the accident and died of cancer years later. “He is the only person who could have had adverse health effects from it.” Haas says the only other people who died in the reactor event were patients on ventilators and other life preserving equipment who died in the evacuation of the area. “If you try to evacuate vulnerable people when the power’s out, it’s a risk that can lead to folks not surviving the evacuation. . . . But there are people who will get on the news and say that thousands or hundreds of thousands of people died, and it’s just wrong.”
Even incorporating all three of those accidents, nuclear is still one of the safest forms of energy per kilowatt hour, says Haas. He calls it “about as safe as wind and solar.” With those, because so many apparatuses have to be built to get the same amount of energy, the accidents or deaths per watt is going to be higher statistically merely from the installation of windmills and solar panels and from simply driving equipment down the highway.
And nuclear power is “dramatically safer” than burning coal, he says, because of the health impacts of coal plant emissions. He calls even the current fleet of nuclear reactors, known as light-water reactors, “amazingly safe.”
Haas has personal experience with nuclear safety because, after earning three degrees at UT, the Corpus Christi native knowingly moved to the most contaminated site in North America, Hanford, Wash., where plutonium for nuclear weapons was made and processed. There he worked at the Pacific Northwest National Laboratory to prevent nuclear weapons proliferation. “I could see the site from my backyard. I knew all the risks and chose to live there. I looked at the risks, and I said, yeah this is absolutely safe.”
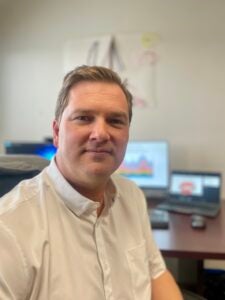
While working there, he visited more than 20 countries doing international security work, but he discovered his favorite part of the job was when he got to train new summer interns. He fed off their excitement about learning new things. When he heard there was a faculty job opening up at his alma mater, he thought, “You know, I could make that my whole job.” He joined the Walker Mechanical Engineering Department in 2016 and teaches undergraduate and graduate courses in nuclear engineering.
***
With all that said about safety, nuclear energy could be made even safer, and here is how:
The fuel in a molten salt reactor starts by taking salt, not too different from table salt, and melting it. At a very high temperature, it flows like water. At this point, uranium is added to the brew and melts with it. When the fuel is put into the reactor, the uranium is bombarded with neutrons kicking off the chain reaction of atomic fission, “going critical,” which generates even more heat. Whatever heat is generated is siphoned away and converted to electricity.
The reason these will be safer than current light-water reactors is they will operate basically at atmospheric pressure instead of within high-pressure systems that can build up steam and explode, as at Fukushima. So even if there were a failure of some kind, there is nothing propelling radioactive material out of the plant and into the environment.
Additionally, the salt wants to be a solid, so if it gets out of its furnace, it simply drops it into a container that’s not being heated and cools into a solid, retaining most of the radioactive material. “It’s not going to be fun to clean that up, but there’s no energy behind it to launch it into the environment.” This is known as “walk-away safe.”
***
Equally vital to the project’s success is driving the cost down. This issue fits hand-in-glove with the safety issue. Because light-water reactors have had to be overbuilt because of safety concerns, they have been incredibly expensive to construct. The South Texas Project took 14 years and about $17 billion (adjusted) to build. “If we can change what the reactor looks like, we can incorporate more inherent safety and bring the cost down so it’s competitive with other sources of energy. If you can make the reactor inherently safe, you make it less expensive. So it’s really an economics argument, not a safety argument.”
This new chapter in nuclear research began not in Austin but in Abilene.
A group of professors at Abilene Christian University had become interested in molten salt reactors and gave a presentation to prospective donors. When they finished, third-generation Midland oilman Doug Robison pulled one of the professors aside and asked, “What would you do if you were fully funded?”
They rolled out a research program with $3.5 million in the form of a donation from Robison, and soon after had a meeting in Washington, D.C., with Department of Energy (DOE) officials. According to Haas, the DOE said in essence, “This is amazing. We need you to be bigger and move faster” — not least because China and Russia are developing the same technology.
They wanted to build a reactor that would jumpstart an industry based on the technology. Though Robison privately funded the research, they needed the DOE to provide the fuel and salt, just like the DOE provides fuel to UT Austin, Texas A&M, and every other university research reactor in the country.
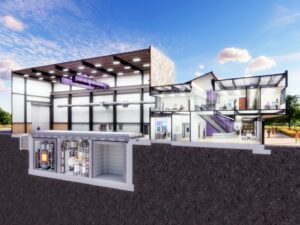
At that point Robison launched the company Natura Resources. “He knew that if you built a program based purely on donations it would stagnate.” They would need to make it profitable and to build hundreds or thousands of these reactors, Haas says.
“As soon as Natura (na-TOO-rah) Resources was founded, they said they definitely needed more help. So they reached out to both UT and A&M.” Georgia Tech is also a partner.
***
UT has been teaching about nuclear energy since 1957, when it began offering the course Nuclear Reactor Operation and Maintenance. In 1960, nuclear engineering courses were offered in Engineering Science and in 1970 the program moved to the Mechanical Engineering Department, where it still resides. In 1963, UT’s first nuclear reactor, a TRIGA, went critical.
- There was a reactor in the basement of Taylor Hall at Speedway and 24th. According to Haas, “In the ’80s, the administration said, ‘We’re going to build a new building. We need a reactor to not be underneath it. They said, ‘We’re either shutting your reactor down or you can have a brand new one out at the Pickle Campus, and the head of the reactor said, ‘We’ll take the brand new one, please.’ So we have the newest research reactor in the United States, and it was completed in 1992. It is older than just about all of our students, and we’re hoping to lose that crown very soon with the reactor coming online in Abilene.”
- The Nuclear Engineering Teaching Laboratory at J.J. Pickle Research Campus in north Austin supports research in health physics, materials science, radioactive waste management, experimental and computational nuclear engineering, homeland security, and nuclear nonproliferation. It is directed by Bill Charlton, who is also a professor in the Walker Department of Mechanical Engineering
- UT also had a fusion reactor, the donut-shaped tokamak, completed in 1971 and operated beneath the Physics, Math and Astronomy Building (then RLM). The last iteration of the Texas Experimental Tokamak was dismantled and shipped to China in 2004.
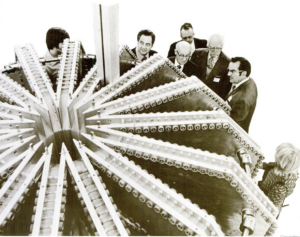
Haas was familiar with the concept of molten salt reactors from graduate school, and in his prior job he understood them from the security side but only knew the basics. When this opportunity arose, he tried to be clear with the ACU team, asking, “You know I’m not a nuclear reactor guy, right? I’m a nuclear security guy.” They said they needed him to join the project.
“Even though nuclear reactors cannot explode like a nuclear bomb, the skills and understanding of physics and chemistry one needs to detect nuclear explosions are exactly the same fundamentals one needs to evaluate reactors to make sure they’re going to be safe,” Haas says.
“We started with a white board that was blank and asked, what are we going to make this reactor look like, and why?” The main thing they knew was that they needed to have a relatively inexpensive reactor that would get through the licensing process at the Nuclear Regular Commission. “If we came in and tried to design the perfect reactor, it was going to take too long and be too expensive. So we said, what is inexpensive in the nuclear realm — which is still very expensive — and will be nearly guaranteed to be licensed as safe by the federal regulators?”
Over the next several years they fleshed out the design and submitted it to the NRC. In September 2024, that construction permit application was approved, and they are now in the process of building the reactor. The best-case scenario for completion is the end of 2026. “That’s entirely based on how long it takes us to get the fuel from DOE,” Haas says.
***
The site of the molten salt reactor is right on the ACU campus. (Haas has lost count of how many times he has driven to Abilene over the last three years; he has his favorite routes based on the time of day and avoiding deer at night.)
The reactor core will be about as big as a refrigerator, and the whole reactor unit will fit onto the back of a flatbed trailer. This is key to the assembly line model the consortium envisions.
“Historically, we build very expensive plants, $10 billion-plus projects that go over budget because a slight delay drives the cost up dramatically and has cascading effects. If we can make each project smaller, we tend to be better at staying on budget and meeting timelines.”
Also, he says we get good at building things when we build a lot of them. “The hope with smaller reactors is that we can build the reactors in an assembly line environment where you maintain the skilled labor.” Most workers won’t have to travel to the site but will do most of the skilled labor at the factory, then install it at the end-use location.
These smaller reactors could provide very localized power for things such as data centers, but, like Legos, they also could be put together to create a single, grid-scale plant that could serve a city.
And the shipping-container size is no coincidence. “One of the reasons Texas is interested in nuclear power is that we want to build those factories here. We want to build the factories to power our own needs, but we also want to export those reactors to the world.”
Molten salt reactors produce a much higher temperature, 650 C instead of 300 C, than current reactors, opening nuclear power to a whole new group of users including petrochemical and plastics refineries. “All of the refiners on the Gulf Coast would love to use nuclear reactors and never have to convert the heat to electricity — just take the heat straight out of the reactor and apply it to the refinery.” This would be three times as efficient as using electricity as an intermediate step
Reactor developers will likely first approach potential customers such as refineries and data centers, consumers of large amounts of electricity willing to pay above market rates, especially for carbon-free energy that is very reliable. Nuclear “checks all of those boxes.” Once these companies in the vanguard of nuclear power start to create enough demand, the price will start to drop such that consumer power will then become an option, deploying reactors for the grid.
Haas says one of the sector’s biggest challenges is shortage in the workforce. “Nobody’s gone into nuclear for years. I went into it for security reasons, not to be a nuclear energy guy. So we’ve got to get more people into it and then retain them.”
***
What if the byproduct of producing electricity was freshwater?
One of the drawbacks to desalination is that it requires a lot of energy, so only those who are desperate for freshwater and have lots of energy, for instance countries in the Middle East, currently use desalination plants. Texas needs more water, and nuclear power can provide that by desalinating seawater and brackish groundwater. When electricity is generated, the waste heat that has to be disposed of anyway can be used to desalinate water by turning the seawater into steam, which then condenses into fresh water.
Moreover, there is some uranium in seawater, so taking in seawater for desalination could double as a uranium harvesting operation. About 5% of the uranium needed to fuel a reactor could be taken out of the water being desalinated.
Even though nuclear energy is based on a mined substance, could it be considered renewable? “Uranium is fairly plentiful on Earth,” says Haas, “and as with oil and gas, every time the price goes up, explorers become more incentivized to look for it, and they find it in high concentrations.” But even if that stopped happening, he says “there are thousands of years’ worth of uranium in the world’s oceans, and we have the technology to pull the uranium out. We don’t do that today because it’s more expensive than finding new uranium on land.
“We could power society as we know it today for thousands of years with uranium, and uranium from the Earth’s crust is constantly replenishing uranium from seawater.”
Another benefit of nuclear power rarely considered is that it can provide isotopes to help treat cancer. There are promising cancer treatments, “but there is such a limited supply of isotopes that we’re basically stuck.” Doctors perform more than 40 million procedures each year that rely on medical isotopes, but most of these procedures are for diagnosing disease rather than treating it. More nuclear reactors could produce more isotopes for life-saving treatments.
***
In the big energy picture, Haas says other technologies could be dramatically expanded, especially natural gas, though he says, “Eventually, we’ll have to get off of natural gas. If we want to limit carbon emissions, then we need to add on carbon capture and sequestration. We could absolutely do that,” adding there are promising technologies to bring the cost of that down. Geothermal is another area of focus he thinks holds a lot of promise. “Battery technology is obvious, but we have a very long way to go until batteries plus wind and solar could become effectively as reliable as these other forms of energy. We’re not close yet.”

“Wind and solar are great to have on top of a reliable energy mix,” he says. But the fact that they are intermittent requires a certain level of storage that is simply not economical right now. “Even in the best places on Earth, it’s not sufficient. We have to have that baseload and dispatchable energy supply, and we learned in Texas during winter storm Uri what it looks like to not have sufficient dispatchable energy. Nobody wants to have that again. We learned what most of the world understands because they live it all the time: when energy goes away, life gets harder,” says Haas.
“At least where we sit today, we still need something like nuclear, geothermal and natural gas to maintain our standard of living.
“If we can make nuclear energy less expensive while maintaining the excellent safety record that it’s had we can provide energy to billions of people who don’t have enough right now, and we can provide more energy to people who already have it.”